The avian blink
The phenomenon of blinking on head turns
John GL Morris and Andrew Duggins
Department of Neurology, Westmead Hospital, University of Sydney
Abstract
It is a long-standing though neglected observation that birds blink when they turn their heads. This leads to the question, first raised more than 80 years ago by Mowrer, of whether blinking has a role to play beyond one of corneal maintenance and protection. A video study with frame by frame analysis of blinking in 226 species of birds from 24 Orders has been done to further explore this question. This shows that birds have two main types of blink, a tonic blink (slow and prolonged) which occurs during preening and when the bird is drowsy, and a phasic blink (rapid and brief) which occurs particularly during head movement. Tonic blinks mainly involve the lower lid, often accompanied by the nictitating membrane and sometimes the upper lid. Phasic blinks are performed by the nictitating membrane in most Orders investigated and by the upper lid and nictitating membrane in three Orders. 540 blink/head turn pairs were analysed using linear regression. The mean blink duration was 132ms and the mean head turn duration was 169ms. The estimated blink/head turn gradient was 0.63, meaning that for every 100ms extra head turn duration there was a 63ms extension of blink duration. The duration of phasic blinks correlated strongly with the duration of head turns (p<0.00001). A case is made that phasic blinks have a function in stabilising vision by excluding images during the rapid early phase of head turns.
​
Introduction
There have been few detailed studies of blinking in birds. One of the earliest and best accounts of the anatomical basis for blinking is provided by Owen (1835). He noted that the nictitating membrane was drawn across the cornea by the combined action of the quadratus and pyramidalis, muscles which lie behind the eyeball. The lower eyelid rose in sleep and was generally more mobile than the upper lid. Exceptions to this were owls and nightjars which blink with their upper lids, while also activating the nictitating membrane.
​
Blount (1927) measured the frequency of blinking in a wide range of mammals and in four species of birds. An ostrich blinked every 1.1 seconds, a parrot every 2.3 seconds and an owl every 11.2 seconds. Relevant to the present study, he noted that in young calves blinking occurs during head movement ‘to prevent unnecessary blurring of the image’.
​
In an anatomical study, Stibbe (1923) contrasted the nictitating membrane blink of birds with that of mammals. In the latter, the membrane is not drawn across the eye by the contraction of muscles. Rather, it lies in a state of elastic tension out of sight on the medial side of the globe, springing across when the eyeball retracts by the action of the retractor bulbi muscle. In cats and dogs, this only occurs during blinking with the eye lids.
​
The present study could be characterised as a belated follow-up to two seminal papers by Mowrer and colleagues (Dunlap and Mowrer 1930)(Mowrer 1933). They noted that the nictitating membrane in birds flicks across the eye, eliminating distinct vision during head movements which they described as quick and jerky like the saccadic eye movements of primates. They postulated that the nictitating membrane prevents images which are blurred as a result of head movement from reaching the retina. The onset of blinking often precedes the onset of head movement and blinking rarely occurs during the stationary phase of head bobbing. Mowrer was aware of Dodge’s seminal study (1900) of suppression of vision during human saccades and made a strong case for blinking providing another means of filtering out unwanted images due to movement.
​
Yet in the years after these publications, Mowrer’s observations and ideas were largely forgotten. For example, Kirsten and Kirsten (1983) studied blink rates in 25 mainly caged birds and speculated that blinking during head movement might be due to stimulation of the vestibular apparatus.
The advent in recent years of high resolution, high speed digital video cameras which work in low light and at high image magnification, and the development of video-editing software which lends itself to frame by frame analysis of what has been recorded, has provided an opportunity to revisit Mowrer’s hypothesis. It is now possible to measure, among other things, the duration of blinks with some accuracy.
​
But how to show that blinking on head turns fulfils the role suggested by Mowrer? The present study confirms that the onset of blinking usually coincides with or precedes the onset of head turn, that the duration of the blink is shorter than that of the head turn and that there is a strong correlation between the duration of the blink and that of the head turn. Moreover, most head turns are accompanied by blinks. Thus vision is blocked for most of the duration of a head turn and is only unblocked as the head movement slows down towards its termination. These findings are strongly supportive of Mowrer’s hypothesis, though not definitive. Why, for example are not all head turns accompanied by blinks and conversely, why do bursts of blinking sometimes occur with the head still? These have still to be explained.
​
Whether blinking simply blocks the image from reaching the retina, or activates a central mechanism for suspending vision as occurs in primates, cannot be answered by this study. This would involve recording from the central visual pathways in a conscious bird while providing a light stimulus, which bypasses the retina, both during and between blinks – as has been done in primates (Gawne and Martin 2002).
​
In the course of testing Mowrer’s hypothesis, an opportunity arose to classify spontaneous avian blinks according to: their duration, which of the three ‘lids’ were involved, and when they tended to occur. Two main types of blink were found. Phasic blinks mainly involve the nictitating membrane, are brief and are seen with the head at rest and during head movement. Tonic blinks mainly involve the lower eyelid, are prolonged and are seen mainly during preening and as a prelude to sleep.
Here, blinking in birds is defined broadly as transient and repetitive closures of the eyes which may involve the upper eyelid, the lower eyelid, the ‘third’ eyelid (the nictitating membrane), or a combination of all three.
​
General methods
Birds were video-ed using a Panasonic Lumix DMC-FZ200 Digital Camera. The zoom on this allowed close up views of the eyes even when the bird was more than 5 metres away. Most filming was done at 25 frames/second and in some circumstances, 100 and 200 frames/second. Birds were video-ed for as long as they remained within view unless they were still, when it was done for a minimum of about a minute. When the opportunity arose, the same bird was often video-ed repeatedly.
​
Wild birds were studied in the Bush and public parks around Sydney, Australia, and to a small extent in public parks in the UK and the Netherlands. No specific permission was required for photography in any of these locations. Captive birds were filmed at Taronga Zoo, Australian Reptile Park and Featherdale Wildlife Park (in and near Sydney); Jurong Bird Park (Singapore); London Zoo and Suffolk Owl Sanctuary (UK); Rotterdam Zoo and Vogelpark Avifauna (Netherlands).
​
The studies involved videoing of the birds without in any way interfering with their activities for example by attracting their attention with calls or using lights or flash. As a zoom lens was used, it was possible to film wild birds at a distance. None of these included protected or endangered species. The rules regarding photography varied from zoo to zoo but in no case was photography banned provided that photographs and videos were not published (without permission) for commercial purposes. As no photographs are included in this publication and as the only access to the birds was the same as for every other visitor, no permission was sought from the zoo authorities. As neither captive nor wild birds were subjected to any interference beyond that of being video-ed from a distance, approval from an Ethics Committee was not sought.
​
Videos were analysed frame by frame using Cyberlink PowerDirector and VLC (VideoLan Client) Media Player video editing software. In the case of blinks associated with head movement, several blinks were compared wherever possible in order to determine the relationship between blink duration and head turn duration. The interval between the onset of blink and head movement was noted. Stills were taken of the whole blink sequence in many cases. An estimate was made of the duration of blink and the duration of the head turn, based on the number of video frames taken up by each. Where multiple head turns were available for analysis, the proportion in which these were associated with blinks was noted. In some birds, only a few blinks and head turns were recorded in the time available. In a few species, no phasic blinks were seen. Data was pooled for all birds on which information on head turns and blinks were available.
​
The opacity of the nictitating membrane was judged by the appearance of the iris and pupil through it, using this grading: Opaque (iris and pupil not visible), Cloudy (iris and pupil seen indistinctly), Clear/transparent (iris and pupil seen clearly).
​
With the exception of raptors, only one eye was usually seen, but where both were visible, note was made of whether blinking was bilateral, synchronous or unilateral in the two eyes. All filming was done during daylight hours. No time limit was set on how long a blink could last but the end of the blink had to be seen for it to be so designated. The results for each species mainly involved a single individual but in some cases, the results of more than one individual are included to show, for example, what happens in drowsiness and preening (which may be difficult to capture in the time available in a single bird).
​
For the analysis of results, birds were grouped phylogenetically into Orders according to the schema provided by Jarvis et al. (2014).
​
Statistical methods
We hypothesised that over repeated measurements within individual birds, or across individuals but within species, there would be a linear relationship between head turn and blink duration. In order to explore the overall association between head turn and eye blink duration, we calculated a Pearson Correlation Coefficient. We further tested the hypothesis using linear regression: treating eye blink duration as the dependent and head turn duration as the explanatory variable, estimating the (blink/ head turn) gradient, and expressing the deviation of this parameter from zero as a t-statistic. Conceivably, an apparent relationship between head turns and eye blinks might reflect between-order or between-species effects, rather than the within-species effect that we aimed to measure. Conversely, a real within-species effect of head turns on eye blink duration might be masked by between group variation. Extending simple regression to a General Linear Model, we were able to capture these and other potential confounds as nuisance covariates.
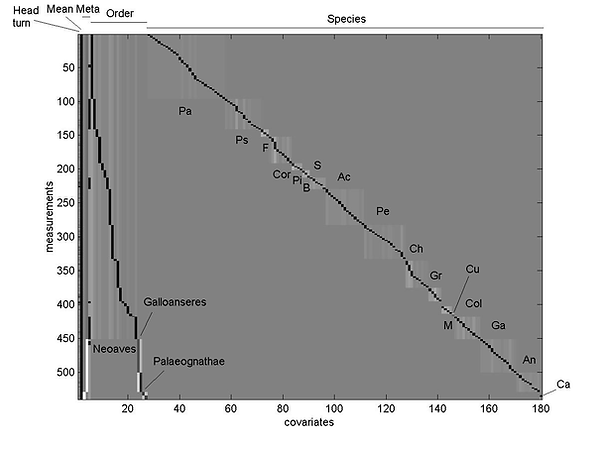
Figure 1
In this image of the design matrix, elements varying from -1 to 1 are illustrated as shades varying from white to black respectively. There is a row for every eye blink duration measurement analysed. There is a column for every covariate, and for each a parameter is estimated so that the sum of columns weighted by respective parameters best models the data. All columns are mean-corrected apart from the second column of all ones (‘Mean’), so that the corresponding parameter estimate becomes the mean of the eye blink data. The explanatory variable of interest is in the first column (‘Head Turn’). All other columns are nuisance covariates, in which 1- or 0-elements identify category membership, prior to mean-correction. The first two ‘Meta’ categories group together similar orders of birds, the third identifies measurements of upper-lid blink duration (usually a joint characteristic of birds within an order or species). ‘Order’ columns identify in turn Neoave, Galloansere and Palaeognathae orders of birds. ‘Species’ columns identify birds belonging to individual species within the following orders: ‘Pa’ = Passeriformes, ‘Ps’ = Psittaciformes, ‘F’ = Falconiformes, ‘Cor’ = Coraciiformes, ‘Pi’ = Piciformes, ‘B’ = Bucerotiformes, ‘S’ = Strigiformes, ‘Ac’ = Accipitriformes, ‘Pe’ = Pelicaniformes, ‘Ch’ = Charadiformes, ‘Gr’ = Gruiformes, ‘M’ = Musophagiformes, ‘Cu’ = Cuculiformes, ‘Col’ = Columbiformes, ‘Ga’ = Galliformes, ‘An’ = Anseriformes, ‘Ca’ = Casuariformes.
Design matrix X, illustrated in Figure 1, defines the statistical model used in the analysis. The matrix has r rows corresponding to the number of eye blink duration measurement in the data, arranged as a column vector y. The main effect of head turn duration is modelled by the column at far left of X (‘Head turn’), the mean eye blink duration by the second column of ones (‘Mean’), and confounding effects by nuisance covariates further to the right. The first 3 of the latter (from left to right) identify membership of meta-categories (‘Meta’): Neognathae, Neoaves and upper lid blinkers respectively. The next 3 blocks of covariates (‘Order’) categorise respectively Neoaves, Galloanseres and then Paleognatheae by membership of specific order. The last 17 blocks of covariates (‘Species’) categorise birds of each order by membership of specific species. Where an order is represented by only one species, a separate species covariate is redundant and has been omitted. No covariate is included for species with just one individual member. All c columns are mean-corrected apart from the second column of ones. Whether a member of a higher-level group is a member (or not) of a lower-level group is in every case represented by a matrix element 1 (or 0), prior to mean-correction within higher-level group, giving the matrix image its block-diagonal appearance.
​
A column vector b of parameter estimates, one for each column of the design matrix, that minimises the residuals e in the matrix equation y = Xb + e is given by b = DXTy where the matrix D is the Moore-Penrose pseudoinverse of the rank-deficient dispersion matrix XTX (i.e. rank(X): k < c). This minimal residual variance, s2, is the sum of squared residual elements, eTe, divided by the degrees of freedom, r – k.
​
The blink/ head turn gradient, the first element b1, has variance D1s2 where D1 is the first element on the principal diagonal of D. We assess the null hypothesis b1= 0 by comparing b1/√ D1s2 with a student’s t distribution with r – k degrees of freedom.
​
The hierarchical structure of X allows us to assess whether inclusion of each additional block of nuisance covariates, corresponding to a further sub-categorisation of birds Meta>Order>Species, has a significant effect on the data. For example, solving as above a reduced design matrix Xred, lacking additional species columns to the right and of lesser rank kred, gives a greater residual sum of squares. The ratio of this additional variance sadd2 to the original variance s2, an F statistic with numerator degrees of freedom k – kred and denominator degrees of freedom r –k, expresses evidence for the inclusion of species columns.
​
General results
Information was gathered on 226 species of bird covering 24 Orders. Given the opportunistic nature of the study, it was not possible to observe all the features of interest in every individual. The number of individuals on which information is available for each type of measure is provided in the figures and tables.
Two types of blink were observed: Phasic blinks, which were brief and rapid; and tonic blinks, which were prolonged, slow and occurred during preening and in drowsiness. The duration of phasic blinks (Bl) and duration of the associated head movement (HT) in 187 species belonging to 20 Orders are shown in Figure 2 and Table S1. In most Orders, the duration of the head turn was longer than that of the blink.
​
In Table S1, the minimum, median and maximum durations of blink (ms) are shown for the same data as Figure 2. Excluding the outliers, the duration of blink in most Orders lay between 40 and 200ms (40ms is an artificial minimum as it represents the duration of a single frame with a video frame rate of 25/second). Caprimulgiformes, Sphenisciformes and Strigiformes had the longest durations of blink, Passeriformes the shortest. Also shown in this Table are the median durations of blink and head turn (ms) together with the ratio of one to the other. The duration of the head turn is shown to be longer than that of the blink in most Orders, the range varying from 0.5 to 1.

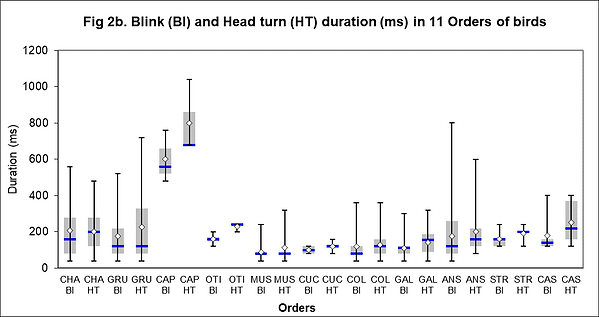
Table S1

Figures 2a&b and Table S1. Duration of blinks (ms) in 20 Orders of birds (Quartile chart: grey box represents 50% of the data; whiskers represent maximum and minimum values; diamond is average and horizontal line is median). Abbreviations and numbers of species in Orders: PAS: Passeriformes (32 species); PSI: Psittaciformes (19 species); FAL: Falconiformes (4 species); COR: Coraciiformes (7 species); PIC: Piciformes (4 species); BUT: Bucerotiformes (5 species); STR: Strigiformes (11 species); ACC: Acciptriformes (16 species); PEL: Pelicaniformes (20 species); SPI: Sphenisciformes (1 species); CHA: Charadriformes (10 species); GRU: Gruiformes (6 species); CAP: Caprimulgiformes (1 species); MUS: Musophagiformes (4 species); CUC: Cuculiformes (2 species); COL: Columbiformes (12 species); Gal: Galliformes (14 species); ANS: Anseriformes (15 species); STR: Struthioniformes (1 species); CAS: Casuariformes (2 species). Strigiformes, Sphenisciformes and Caprimulgiformes stand out as having the longest durations of blink. Passeriformes was among the Orders with the shortest duration of blink.
Using the same data set as in Figure 2a&b, the correlation between the duration of head turn and the duration of blink was calculated: R=0.835282. The R2 value of 0.8 implies that 80% of the variance in eye blink duration is attributable to variable head turn duration. However, this result does not distinguish a between-order or between-species effect from a within-species effect (see Statistical Methods).
The distribution of the intervals between the onsets of blink and head turn from the same data set as in Figure 2 is shown in Figure 3. In the majority, the blink onset occurred in the same frame (0ms) or the previous frame (40ms) as the onset of the head turn. In a small number of species, the head turn preceded the blink by 1 frame (-40ms).
​
In some individuals, enough blinks and head turns were recorded during the observation period to provide an estimate of the concordance between the two. As shown in Figure 4 and Table S2, the median concordance between head turns and blinks was 84.5%, but concordance varied greatly between species and probably within individuals. For example, a Boobook owl (Ninox novaeseelandiae), blinked five times consecutively on head turns and then did not blink for the next five head turns, as though the link between the two could be switched on or off. The head turns in the two sequences appeared to be similar in velocity and duration.
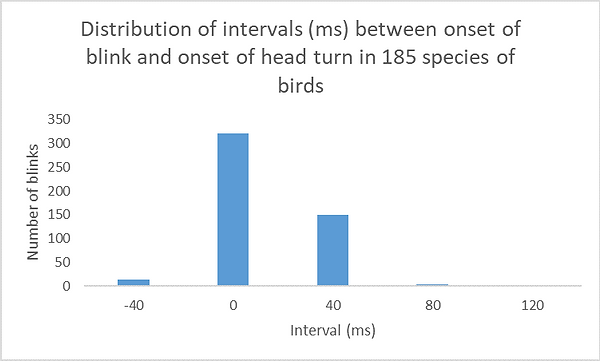
Figure 3. Distribution of intervals between the onset of blink and the onset of head turn (ms) in 185 species of birds. In the majority, the blink onset occurred in the same frame (0ms) or the previous frame (40ms) as the onset of the head turn. In a small number of species, the head turn preceded the blink by 1 frame (-40ms).
Eye movements were minimal in most species studied. In a few, mainly those with large bills, a moderate range of eye movements was visible. Phasic blinks linked to eye movements (Table S2) were seen in several Hornbills, Grey goshawk (Accipiter novaehollandiae), Australian pelican (Pelecanus conspicillatus), Royal spoonbill (Platalea regia), Nankeen night heron (Nycticorax caledonicus) and White-faced heron (Egretta novaehollandiae), and some cockatoos. In two species, the concordance between eye movements and blinks was in the range of 40-55%.

Figure 4. Concordance between head movements and blinks in 20 species of birds (Quartile chart: grey box represents 50% of the data; whiskers represent maximum and minimum values; diamond is average and horizontal line is median). The median concordance is 84.5%, minimum 9% and maximum 100%.

Table S2. Concordance between head movements and blinks in 20 species of birds and between eye movements and blinks in 2 species. The concordance varies between 9 and 100%.
While birds did not always blink during a head turn, the converse was also true. Phasic blinks were seen with the head stationary in many species. These were usually brief (40ms) and occasionally occurred in clusters, for example, ten blinks in 15 seconds in a female mallard (Anas platyrhynchos), 13 blinks in 22 seconds in a kelp gull (Larus dominicanus), and 14 blinks in 14 seconds in a Eurasian spoonbill (Platalea leucorodia).
​
Prolonged blinks with the head both still and moving were seen associated with rapid oscillations of the eyeball, only visible at high video frame rates. Videos of these were shown to Professor Jack Pettigrew who identified them as saccadic oscillations (Pettigrew 1990).
​
The phasic blink type, tonic blink type and opacity of the nictitating membranes in the 23 Orders are shown in Table 1. In most Orders, phasic blinks were done by the nictitating membrane, the three exceptions being Columbiformes, Psicattiformes and Strigiformes where the upper lid was also involved. The phasic blink of the Tawny frogmouth was intermediate between a nictitating membrane and upper lid blink with partial lowering of the upper lid and crossing of the nictitating membrane.

Table 1. Phasic blink type, tonic blink type and opacity of the nictitating membranes in 25 Orders of birds. (Abbreviations: Nict, Nictitating membrane blink; UL, Upper lid blink). In all but three Orders, the phasic blink was a nictitating membrane blink. The lower lid was involved in all tonic blinks. The nictitating membrane was clear in only 4 Orders.
The uniformity in phasic blink type within Orders was good but not perfect. For example, all species of Passerifomes used nictitating membrane blinks except the Eurasian skylark (Alauda avensis) which had upper lid blinks. Galliformes also had nictitating membrane blinks with the exception of the king quail (Coturnix chinensis) and Brown quail (Coturnix ypsilophora) . Interestingly, the painted button quail (Turnix varius) which had previously been included under Galliformes, but is now under Charadriformes (nictitating membrane blinkers), had nictitating membrane blinks. The distinction between nictitating membrane blinks and upper lid blinks was not absolute. In many species with nictitating membrane blinks, minimal lowering of the nasal portion of the upper lid was seen. In Columbiformes, Psicattiformes and Strigiformes, nictitating membrane blinks were never seen on their own i.e. without upper lid involvement, but in some species, for example in the sulphur crested cockatoo (Cacatua galerita), the lowering of the upper lid was minimal in some blinks.
​
The opacity of the nictitating membrane varied markedly within and between Orders. It was clear in most species of Anseriformes and Charadriiformes. Vascular markings at right angles to the axis passing through the canthi were seen in 14 species mainly comprising storks, cranes, stone-curlews and ibis.
​
Tonic blinks nearly always involved the lower lid, accompanied on occasion by the upper lid and nictitating membrane. They were seen during preening and when the bird was still and apparently sleepy. The lower lid would rise slowly over half a second or longer. In drowsiness, the upper lid might then start to fall and the two lids would rise and fall in unison, often providing a glimpse of the nictitating membrane at the times when they were apart. Not uncommonly, a tonic blink would superimpose itself on a phasic blink, greatly prolonging its duration. As discussed above, when 100 or 200 frames/sec video was used, tonic blinks were sometimes seen to be associated with rapid oscillations of the eyeball. In some species, the lower lid appeared to be markedly thin.
​
The time course of the nictitating membrane blink was measured in a few species while videoing at high frame rates. The time taken for the membrane to be drawn across the eye in an Asian black-necked stork, or Jabiru (Ephippiorhynchus asiaticus) was 30ms compared with 60ms for it to recoil. In a grey heron (Ardea cinerea), contraction was slower than recoil, the comparable times being 50ms and 30ms respectively.
In some species, particularly in the smaller passerines, the head turn was so rapid as to preclude visualising the blink.
​
While many birds walked during the course of being videoed, this was often accompanied by other behaviours such as pecking, head turns and courtship rituals. Satisfactory uninterrupted videos of gait were obtained in a carrion crow (Corvus corone), Eurasian magpie (Pica pica), crested pigeon (Ocyphaps lophotes), a number of feral pigeons (Columba livia domestica) and a magpie-lark (Grallina cyanoleuca). In all of these, blinks were observed during the ‘head-thrust’ phase, usually at the onset, and never in the ‘head-stationary’ phase of head bobbing. That said, by no means all head-thrusts were accompanied by blinks.
​
The frequency of phasic blinks of a number of Orders of birds is shown in Figure 5 and Table S3. The frequency was highest in Passeriformes and lowest in Strigiformes; most species across the Orders blinked at a rate of about 20/second though the range was wide. Tonic blinks only occurred with drowsiness and during preening, so measures of frequency meant little.
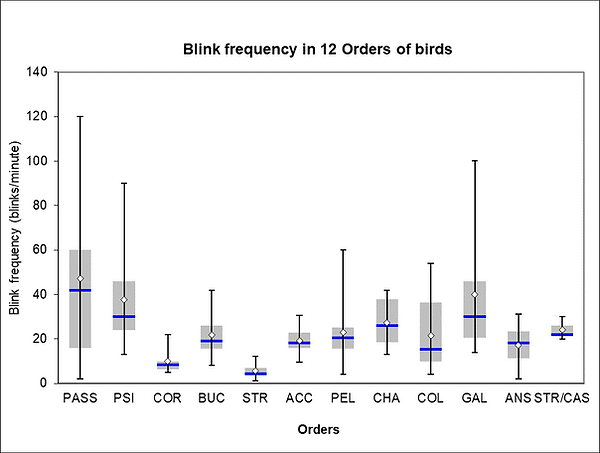
Figure 5. Blink frequency in 12 Orders of bird (Quartile chart: grey box represents 50% of the data; whiskers represent maximum and minimum values; diamond is average and horizontaline is median). Abbreviations and numbers of species studied: PASS: Passeriformes (21); PSI: Psittaciformes (15); COR: Coraciiformes (6); BUC: Bucerotiformes (6); STR: Strigiformes (11); ACC: Acciptriformes (14); PEL: Pelicaniformes (20); CHA: Charadriformes (9); COL: Columbiformes (11 species); GAL: Galliformes (11); ANS: Anseriformes (5); STR/CAS: Struthioniformes (1)/CAS: Casuariformes (2). The blink frequency was highest in Passeriformes and lowest in Strigiformes. The numerical data for this plot is in Table 5 in Supporting Information.

Table S3. Blink frequency (blinks/minute): median, minimum and maximum, in 12 Orders of bird. Abbreviations and numbers of species studied: PASS: Passeriformes (21); PSI: Psittaciformes (15); COR: Coraciiformes (6); BUC: Bucerotiformes (6); STR: Strigiformes (11); ACC: Acciptriformes (14); PEL: Pelicaniformes (20); CHA: Charadriformes (9); COL: Columbiformes (11 species); GAL: Galliformes (11); ANS: Anseriformes (5); STR/CAS: Struthioniformes (1)/CAS: Casuariformes (2). The blink frequency is Passeriformes is nearly tenfold that of Strigiformes.
We analysed 540 eye blink duration measurements using a design matrix X with rows r = 540, columns c = 180 and rank k = 172 (Figure 6). The estimated blink/head turn gradient b1was 0.63, meaning that for every 100ms extra head turn duration there was a 63ms extension of blink duration. The fitted main effect of head turn on eye blink duration is plotted against uncorrected head turn duration in Figure 6.

Figure 6
For every actual eye blink measurement, a fitted response (in red) and adjusted response (in blue) is plotted against the corresponding (uncorrected) head turn duration. After estimation of the model, a fitted response is calculated as: (deviation of the corresponding head turn duration from the mean)* b1 + (mean blink duration), where b1 is the estimate of the blink/head turn gradient, 0.63. An adjusted response, an eye blink duration adjusted for the effect of all nuisance covariates, is the sum of the corresponding fitted response and element of residual vector e. The fitted responses follow a line of best fit of gradient b1 through the adjusted responses. We infer from the statistic described in the text the significance of the deviation of this gradient from zero.
Evidence against a null effect of species covariates on blink duration was calculated as F = 2.77, with numerator 148, denominator 368 degrees of freedom, p < 0.00001.
​
Discussion
Two main types of blink were seen:
Phasic blinks
Phasic blinks are brief, rapid and occur during head movement and also with the head still. The commonest form, the nictitating membrane blink, primarily involves the nictitating membrane, and can be unilateral or bilateral, synchronous or asynchronous. There is no visible retraction of the eyeball. While the nictitating membrane is drawn across the eye by contraction of muscles, it depends on elastic recoil for its return. This is highly effective, contraction of the nictitating membrane in the grey heron, for example, taking 50ms compared with 30ms for the recoil. In many species across most Orders, the nictitating membrane blink was associated with minimal lowering of the nasal portion of the upper lid (eg whistling kite (Haliastur sphenurus), banded lapwing (Vanellus tricolor), cattle egret (Bubulcus ibis), jabiru).
​
In the upper lid blink, the upper lid rapidly lowers to the level of the pupil or below. This was seen mainly in three Orders: Columbiformes, Psittaciformes and Strigiformes. Where the upper lid failed to close completely, it was usually possible to see the nictitating membrane also crossing the eye. In the Orders with upper lid blinks, nictitating membrane blinks were never seen in isolation; this is similar to cats and dogs where the nictitating membrane only emerges in the setting of a lid blink. The rapidity with which the upper lid is lowered suggests that the muscle fibres involved (in orbicularis oculi) are striated as is the case for the muscles which operate the nictitating membrane.
​
The average duration of blink in owls in our study was substantially longer than other Orders, with the exception of the closely related Tawny frogmouth (Podargus strigoides), and the Humboldt penguin (Spheniscus humboldti). The long duration of blinks in owls may have been influenced by the fact that they were filmed during the day and were often still and sleepy. Changes in the velocity, amplitude and frequency of blinking due to drowsiness have been the subject of a number of reports in human studies (Häkkänen et al. 1999). A study of owls at night using infra-red video would resolve this issue.
​
Phasic blinks and head turns
Phasic blinks of both types most commonly began just before or at the onset of a head turn. Pooling the results of all Orders, there was a strong correlation between the duration of the head turn and the duration of the blink. This, however, might reflect between-order or between-species effects. A real within-species effect of head turns on eye blink duration might also be masked by between group variation. Linear regression analysis was therefore performed and the correlation confirmed.
The blink rarely lasted as long as the head turn. The mean blink duration was 132ms and the mean head turn duration was 169ms. The estimated blink/head turn gradient was 0.63, meaning that for every 100ms extra head turn duration there was a 63ms extension of blink duration. Head turns were more often than not accompanied by a blink (median concordance 84.5%) but this varied greatly between and probably within individuals.
​
Could the association between head turns and blinks result from movement of air across the cornea? This is unlikely, for the blink often began before the onset of the head turn. Whatever the trigger for the blink, one likely consequence is protection of the eye during the head turn. Mowrer and colleagues (Dunlap and Mowrer 1930)(Mowrer 1933) have given reasons for supposing that this is not the only function of this type of blink. During rapid head turns, images traverse the retina at a rate beyond the capacity of the retinal photoreceptors to respond adequately (Land and Nillson 2012). This has been recognised in mammals such as ourselves, where the situation arises not so much from head as eye movement. Voluntary eye movement in a person, for example reading a book, is achieved by a series of high velocity jerks – saccades - as the eyes jump from one set of words to the next (Yarbus 1967). At the moments of fixation, when the eyes pause on the words, the image is clear. In between, the image is blurred. Does this matter? Presumably it does, for the human central visual system has evolved an extraordinary, though poorly understood system for excluding these blurred images from consciousness. This is known as saccadic suppression of vision (Ross et al. 2001). Even more impressive, is that we are unaware of any gaps in vision resulting from this suppression. Visual suppression has also been demonstrated when we blink (Volkmann et al 1980). Both saccadic and blink suppression of vision are thought to provide continuity and thereby stability of vision by eliminating blurred images and gaps.
Birds like humans rely heavily on their visual sense for survival. The powers of acuity of vultures and other large raptors even exceed ours (Reymond 1985), reflecting the demands made on their visual system for example in avoiding obstacles or finding perches while flying, or detecting prey at great distances. It would be surprising therefore, if they did not also have a system for filtering out blurred images and 'filling' the resulting gaps to provide continuity of vision.
​
When observing birds at close quarters, the similarity between their head movements and our eye movements is striking. The head jerks rapidly from one point of fixation to the next, in what Mowrer (Mowrer 1933) called saccadic head movements. But there are differences between these head movements and saccadic eye movements. In the latter, the movement always has an abrupt onset, a maximum fixed velocity (depending upon the amplitude of the saccade) which cannot be voluntarily modified, and an abrupt end (Leigh and Zee 2006). By contrast, avian head jerks vary greatly, beginning and ending abruptly or gradually. Most often however, the head movement tails off slowly after the blink has finished.
​
During the period of maximum velocity of head movement, images are excluded from the retina by a blink. That the blink ends before the head turn may mean that in the slower phase at the end of the movement, there is no benefit from shutting out images which are no longer blurred. In the case of a nictitating membrane blink, the extent to which such images are shut out will depend on the opacity of the membrane. In the species studied in which the nictitating membrane was opaque, no images would reach the retina during a blink. In upper lid blinks these images would also be excluded. But what of the species where the nictitating membrane was cloudy and the few where it was clear? While the membrane might blur the edges of a shape, it would not entirely shut out the image. Even if it did, birds would still have to deal with frequent 'blanks' during blinking, which would interfere with the continuity of vision. It may be, that as with humans, the act of blinking in birds, induces central suppression of vision. To prove this would require the insertion of recording electrodes along the central visual pathways and then stimulation of the retinae by a light source which bypassed the eyelids. The response of the visual system to light in a conscious bird could then be tested during and between blinks as has been done in primates (Gawne and Martin 2002).
​
Side to side peering and blinks
Not all head movements in birds were associated with blinks. A great horned owl (Bubo virginianus) made side to side peering movements of its head as the bird appeared to be estimating the distance of its next perch. When the head is moved, the retinal images of objects near to the eye are displaced more quickly and to a greater extent than are those of more distant objects (Kral 1998). This provides a gauge of distance that is used throughout the animal kingdom (Horridge 1986).
​
Phasic blinks and head bobbing
Blinking in birds has also to be discussed in the context of head bobbing. Humans and some birds have evolved similar strategies for dealing with a moving landscape. While sitting looking out of a moving train window, our eyes fixate on an object, say a house, and hold onto it by allowing our eyes to follow it. As the object moves out of sight, our eyes jump to the next object on which to fixate, using a saccade. This alternation between smooth pursuit and rapid saccades causes a saw-tooth pattern of eye movement - 'optokinetic nystagmus' (Leigh and Zee 2006). Some birds, with their limited range of eye movements, have evolved a similar system but using head movements (Dunlap and Mowrer 1930). While walking or swimming, the bird has alternating periods in which the head does not move relative to the landscape though it's body keeps going (‘hold phase’), followed by a catch up period during which the neck, which has coiled up to hold the head still, unfolds (‘thrust phase’). During the hold phase, the eye(s) can fixate on a stationary landscape for a moment. During this phase, none of the birds we videoed blinked. Phasic blinking was commonly (but not always) seen in the thrust phase of gait. Dunlap and Mowrer (1930) also described nictitating membrane blinks only occurring during the thrust phase though this was not confirmed in a later study by Bangert (1960).
​
In a comprehensive review, Necker (2007) listed the Orders of birds which exhibit head-bobbing (Columbiformes, Galliformes, Gruiformes, Ciconiiformes) and noted that it is only seen in birds which walk on the ground, and then mainly when taking large strides. He concluded that that the primary function of head-bobbing relates to vision. He also discussed the many Orders which do not exhibit head bobbing. Small birds hop, an activity which involves rapid head and body movement followed by a brief period in which the head is stationary. Raptors, which have forward facing eyes, do not bob their heads as the angular velocity of the passing scene is low for objects ahead. (Whiteside 1967).
​
Phasic blink with pecking
A particular form of blinking was noted in the Zebra dove (Geopelia striata). Here the bird appeared to identify a food target, then blinked, completely covered the eye with the upper lid (both eyes were not visible) before beginning the peck. Assuming that both eyes were shut, the resulting peck was directed from memory, as the target was no longer in sight. This happened repeatedly. Presumably this type of blink has evolved as a way of protecting the eyes from injury during pecking.
​
Phasic blink with the head still
Phasic blinks also occurred when the head was still. These presumably fulfil the function of clearing away debris and lubricating the cornea with the secretions of the Harderian and to a lesser extent the lacrimal glands (Bayon et al. 2007). Sometimes, these occurred in rapid bursts lasting for a few seconds without any obvious physical stimulus such as wind or dust. In one female grey lag goose (Anser anser)) guarding her young, rapid blinking may have reflected anxiety. In a male grey lag goose with his head at an aggressive vertical angle, blinking all but ceased. Like other Orders (see below), blinking in birds may be affected by mood and state of arousal. It may also be a means of social signalling (Guilleman et al. 2012).
Some nictitating membrane blinks were prolonged, lasting for hundreds of milliseconds, and associated with oscillations of the eyeball that were so rapid they could only be seen on high speed video. These were often combined with elevation of the lower lid. The eye movements are saccadic oscillations which have been shown to increase perfusion of nutrients to the retina by shaking the pecten oculi (Pettigrew et al. 1990).
​
Tonic blinks
These were mainly lower lid blinks. As the bird became drowsy, the lower lid slowly rose, often rising and falling, sometimes in concert with the upper lid, eventually covering the eye completely. Lowering of the upper lid during tonic blinks often coincided with activation of the nictitating membrane, which then retracted as the upper lid rose again (though the lower lid was still partially elevated). Tonic blinks involving the lower lid were also seen during preening, both when self-preening and when being preened by another bird. The slow rise and fall of the lower lid are best explained by contraction of smooth muscle fibres of orbicularis oculi and the inferior tarsal muscles respectively, under the influence of autonomic nerves.
​
In some species, for example, the great hornbill (Buceros bicornis), the lower eyelids were markedly thin allowing changes in illumination to reach the retina. This might allow the shadow of a predator to be detected even with the eyes closed.
​
In summary, lower lid blinks appear to be a prelude to sleep as well as having a protective function, guarding the eye against injury during preening. The Ural owl (Strix uralensis), tawny frogmouth, Nicobar pigeon (Caloenas nicobarica), Zebra finch (Taeniopygia guttata and sulphur-crested cockatoo were the only species observed to lower their upper lids in drowsiness.
​
Evolutionary considerations
Eye movements are thought to have evolved in the fish ancestors of all vertebrates as a means of keeping images fixed on the retina long enough for the retinal photoreceptors to respond (Land and Nilsson 2012)(Walls 1962). At some stage in their evolution, most birds began to phase out eye movements and came to rely more on head movements to achieve these ends. This was probably a consequence of their adoption of flight with its additional requirements on vision and bone weight. Birds have bigger eyes relative to their body size than mammals (Land and Nilsson 2012). This improves resolution by providing a longer focal length, so that the angle between photoreceptors is as small as possible. A larger lens also reduces diffraction blur. In the case of nocturnal birds like owls, a larger eye improves sensitivity by allowing more light to enter. Having larger eyes and at the same time smaller, lighter bones, placed a premium on space within the skull for the orbits. As a result, there is little room within the orbits for muscles to move the eyes.
​
Saccadic eye movements tend to be very fast (Leigh and Zee 2006), minimising the time taken for the eyes to re-fixate, and it would be hard for a large heavy head to match this. With a small, light head and a flexible neck capable of rapid turns, birds had less need for a full range of eye movements. It is noteworthy that in the present study, the birds which had retained a visible range of eye movements all had large and perhaps unwieldy beaks (hornbills, pelicans, herons, aracaris).
In a study of a human patient with no eye movements due to congenital fibrosis of the extra-ocular muscles, Gilchrist and colleagues (1998) found that saccadic eye movements had been largely replaced by saccadic head movements with good preservation of visual function. They concluded that the saccadic orientating system in gaze, so essential to vision, is the same for eye and head movement. If this is also true in birds, the transition from saccadic eye movements to saccadic head movements would have been facilitated.
​
In most Orders, phasic blinks were performed by the nictitating membrane but even in these it was common to see slight lowering of the nasal part of the upper lid during a nictitating membrane blink. Perhaps this represents a remnant of a time when these species had upper lid blinks. For the three Orders with upper lid phasic blinks, having the additional thickness of the upper lid when blinking would provide more protection from injury. This might be useful in an owl with a struggling prey but is hard to see an adaptive benefit in the other two Orders. Pigeons mainly feed off seed on the ground or fruit in the trees, depending on the species. Parrots are also seed and fruit eaters in the main. The benefit is also hard to see in nectar-consuming hummingbirds which also appear to have an upper lid blink (Attenborough 2015). If no particular adaptive advantage appears to accrue to these Orders from having evolved this type of blink why does it happen? Gould and Lewontin (1978) dealt with this issue in their celebrated essay; the changes could have been secondary to some other useful (but unknown) adaptive change.
​
In conclusion, it is proposed that two types of blink occur in birds. Tonic blinks protect the eyes during preening and sleep. Phasic blinks mainly occur during head movement and, in addition to their accepted role in corneal maintenance, exclude blurred images either directly or more likely by activating central suppression of vision and thereby providing visual stability.
​
References
Attenborough, D. 2015. Conquest of the Skies. Episode 3
Bangert, H. 1960. Untersuchungen zur Koordination der Kopf und Beinbewegungen beim Haushuhn - Z Tierpsychologie. 17:143– 164
Bayon, A., Almela, R.M. and Talavera, J. 2007. Avian ophthalmology. EJCAP. Vol. 17 - Issue 3
Blount, W.P. 1927. Studies of the movement of the eyelids of animals: blinking - Exp Physiol. 18: 111-125
Dodge, R. 1900. Visual perception during eye movement - Psychol Rev. 7:454-465
Dunlap, K., Mowrer O.H. 1930. Head movements and eye functions of birds - Journal of Comparative Psychology. 11:99-113.
Gawne, T.J. and Martin, J.M. 2002. Responses of Primate Visual Cortical Neurons to Stimuli Presented by Flash, Saccade, Blink, and External Darkening - Journal of Neurophysiology. 5:2178-2186
Gilchrist, I.D. et al. 1998. Using the eye–movement system to control the head - Proc Biol Sci. 265(1408):1831-6.
Gould, S.J. and Lewontin, R.C. 1978. The Spandrels of San Marco and the Panglossian Paradigm: A Critique of the Adaptationist Programme - Proc Roy Soc London, Series B. 205:581-598
Guillemain, M., Fouques, C. and Figuero, J. 2012. Consistent contrast between eyelid and iris brightness supports a role for vigilance signalling in ducks - Ibis. 154: 461-467.
Häkkänen et al. 1999. Blink duration as an indicator of driver sleepiness in professional bus drivers - Sleep. 22:798-802.
Horridge, G.A. 1986. A theory of insect vision: velocity parallax - Proc R Soc Lond. B229:13-27
Jarvis, E.D. et al. 2014. Whole-genome analyses resolve early branches in the tree of life of modern birds - Science. 346:1320-1331.
Kral, K. 1998. Side-to-side head movements to obtain motion depth cues: a short review of research on the preying mantis - Behavioural Processes. 43:71-77
Kirsten, J. and Kirsten, E.B. 1983. Spontaneous blink rates of birds - Condor. 85: 92-93
Land, M.F. and Nilsson, D-E. 2012. Animal Eyes. 2nd edn. - Oxford University Press.
Leigh, R.J. and Zee, D.S. 2006. Neurology of Eye Movements. 4th Ed. - Oxford University Press
Mowrer, O.H. 1933. A comparison of the visual function of nictitation and blinking - Journal of Comparative Psychology. 15:75-94
Necker, R. 2007. Head-bobbing of walking birds - J Comp Physiol A. 193:1177–1183
Owen, R. 1835. In: Todd, R.B. (ed), Cyclopedia of Anatomy and Physiology - Longman, Brown, Green.
Pettigrew, J.D., Wallman, J. and Wildsoet, C.F. 1990. Saccadic oscillations facilitate ocular perfusion from the avian pectin - Nature. 343:362-3.
Reymond, E. 1985. Spatial visual acuity of the eagle Aquila audax: a behavioural, optical and anatomical investigation - Vision Research. 25: 1477–1491
Ross et al. 2001. Changes in visual perception at the time of saccades - Trends Neuroscience. 24:121-131
Stibbe, E.P. 1928 A comparative study of the nictitating membrane of birds and mammals - J Anat. 62:159-176
Volkmann, F.C., Riggs, L.A. and Moore, R.K. 1980. Eye blinks and visual suppression - Science. 207: 900-902
Walls, G.L. 1962. The evolutionary history of eye movements - Vision Res. 2:69-80
Whiteside, T.C.D. 1967. The head movements of walking birds - J Physiol. 188(2):3P1-32P
Yarbus, A.L. 1967. Eye movements and vision. - Plenum Press.
​
Acknowledgements
Professor Jack Pettigrew identified the saccadic oscillations seen in some birds. Professor Andrew Roberts, Professor Andrew Beattie, Dr Donald Morris, Dr Jonathan Leicester, Dr Richard Malik, Dr Carl Parsons and Dr Alan Freeman provided much appreciated help in the preparation of this manuscript. Dr Kate Blodley and Falk Wicker checked the identities of the bird species.
There are no sources of conflict of interest.
Appendix
Orders [25] and species [226] of birds studied in the order in which they are thought to have arisen from most recent to most ancient (9):
Neognathae, Neoaves
Passeriformes
(36 species)
Black-naped oriole (Oriolus chinensis), Green figbird [Timor figbird] (Sphecotheres viridis), Australasian figbird (Sphecotheres vieilloti), Apostlebird (Struthidea cinerea), Azure-winged magpie (Cyanopica cyanus), Blue-faced honeyeater (Entomyzon cyanotis), Lewin's honeyeater (Meliphaga lewinii), Noisy miner juvenile (Manorina melanocephala), Carrion crow (Corvus corone), Eurasian magpie [common magpie] (Pica pica), Western jackdaw (Corvus monedula), Pied currawong (Strepera graculina), Eurasian skylark (Alauda arvensis), Chiming wedgebill (Psophodes occidentalis), Common hill myna (Gracula religiosa), Emerald starling (Lamprotornis iris), Violet-backed starling (Cinnyricinclus leucogaster), Common starling (Sturnus vulgaris), Green catbird (Ailuroedus crassirostris), Satin bowerbird (Ptilonorhynchus violaceus), Magpie-lark (Grallina cyanoleuca), Noisy pitta (Pitta versicolor), Saffron finch (Sicalis flaveola), White-lined tanager (Tachyphonus rufus), House sparrow (Passer domesticus), Violaceous euphonia (Euphonia violacea), Diamond firetail (Stagonopleura guttata), Zebra finch (Taeniopygia guttata), Welcome swallow (Hirundo neoxena), Chestnut-breasted mannikin (Lonchura castaneothorax), Orange-headed thrush (Geokichla citrina), Common blackbird (Turdus merula), Pekin robin [Red-billed leiothrix] (Leiothrix lutea), Red-billed blue magpie, (Urocissa erythrorhyncha), White-browed woodswallow (Artamus superciliosus), Ashy woodswallow (Artamus fuscus), Splendid sunbird (Cinnyris coccinigastrus), White-rumped shama (Copsychus malabaricus)
Psittaciformes
(21 species)
Red-tailed black cockatoo (Calyptorhynchus banksii), Yellow-tailed black cockatoo (Calyptorhynchus funereus), Sulphur-crested cockatoo (Cacatua galerita), Baudin's black cockatoo (Calyptorhynchus baudinii), Galah (Eolophus roseicapilla), Cockatiel (Nymphicus hollandicus), Budgerigar (Melopsittacus undulatus), Scaly-breasted lorikeet (Trichoglossus chlorolepidotus), Major Mitchell's [Leadbeater's cockatoo or pink] cockatoo (Lophochroa leadbeateri), Eclectus parrot (Eclectus roratus), Australian king parrot (Alisterus scapularis), Princess parrot (Polytelis alexandrae), Rainbow lorikeet (Trichoglossus haematodus), Turquoise parrot (Neophema pulchella), Eastern rosella (Platycercus eximius), Crimson rosella (Platycercus elegans), Long-billed corella (Cacatua tenuirostris), Green-winged macaw (Ara chloropterus), Red-winged parrot (Aprosmictus erythropterus), Sun conure (Aratinga solstitialis), Superb parrot (Polytelis swainsonii)
Falconiformes
(4 species)
Southern caracara [Crested caracara] (Caracara plancus), Australian kestrel [Nankeen kestrel] (Falco cenchroides), Little eagle (Hieraaetus morphnoides), White bellied sea eagle (Haliaeetus leucogaster)
Coraciiformes
(7 species)
Blue-bellied roller (Coracias cyanogaster), Dollarbird (Eurystomus orientalis), Blue-winged kookaburra (Dacelo leachii), Laughing kookaburra (Dacelo novaeguineae), Collared kingfisher (Todiramphus chloris), Ruddy kingfisher (Halcyon coromanda), Northern carmine bee-eater (Merops nubicus)
Piciformes
(3 species)
Bearded barbet (Lybius dubius), Fire-tufted barbet (Psilopogon pyrolophus), Green araçari (Pteroglossus viridis)
Bucerotiformes
(8 species)
Great hornbill [great pied hornbill, great Indian hornbill] (Buceros bicornis), Blyth's hornbill [Papuan hornbill] (Rhyticeros plicatus), Von der Decken's hornbill (Tockus deckeni), White-crowned hornbill [white-crested hornbill, long-crested hornbill] (Berenicornis comatus), Rhinoceros hornbill (Buceros rhinoceros), Silvery-cheeked hornbill (Bycanistes brevis), Southern ground hornbill (Bucorvus leadbeateri), Visayan hornbill (Penelopides panini)
Strigiformes
(14 species)
Barking owl (Ninox connivens), Barn owl (Tyto alba), Southern boobook [morepork] (Ninox novaeseelandiae), Eastern grass owl (Tyto longimembris), Eurasian eagle-owl (Bubo bubo), Great grey owl (Strix nebulosa), Great horned owl (Bubo virginianus), Northern hawk-owl (Surnia ulula), Little owl (Athene noctua), Powerful owl (Ninox strenua), Rufous owl (Ninox rufa), Snowy owl (Bubo scandiacus), Northern white-faced owl (Ptilopsis leucotis), Indian eagle-owl (Bubo bengalensis), Ural owl (Strix uralensis)
Accipitriformes
(16 species)
Bald eagle (Haliaeetus leucocephalus), Bateleur (Terathopius ecaudatus), Black kite (Milvus migrans), Wallace's hawk-eagle (Nisaetus nanus), Black-breasted buzzard [kite] (Hamirostra melanosternon), Brahminy kite [red-backed sea-eagle] (Haliastur indus), Common buzzard (Buteo buteo), Grey goshawk (Accipiter novaehollandiae), Griffon vulture (Gyps fulvus), Harris's hawk (Parabuteo unicinctus), Palm-nut vulture [vulturine fish eagle] (Gypohierax angolensis), Whistling kite (Haliastur sphenurus), Secretary bird (Sagittarius serpentarius), White-headed vulture (Trigonoceps occipitalis), King vulture (Sarcoramphus papa), Turkey vulture (Cathartes aura)
Pelicaniformes
(28 species)
Hamerkop (Scopus umbretta), Australian pelican (Pelecanus conspicillatus), Great white pelican (Pelecanus onocrotalus), Peruvian pelican (Pelecanus thagus), Dalmatian pelican (Pelecanus crispus), Black-crowned night heron (Nycticorax nycticorax), Glossy ibis (Plegadis falcinellus), Grey heron (Ardea cinerea), White-faced heron (Egretta novaehollandiae), Pied heron (Ardea picata), Eurasian spoonbill [common spoonbill] (Platalea leucorodia), Royal spoonbill (Platalea regia), Great egret (Ardea alba), Nankeen night heron (Nycticorax caledonicus), Abdim's stork (Ciconia abdimii), Northern bald ibis [hermit ibis or waldrapp] (Geronticus eremita), Australian white ibis (Threskiornis moluccus), Scarlet ibis (Eudocimus ruber), Woolly-necked stork (Ciconia episcopus), Black-necked stork [Jabiru] (Ephippiorhynchus asiaticus), Black stork (Ciconia nigra), Painted stork (Mycteria leucocephala), Marabou stork (Leptoptilos crumeniferus), Andean Condor (Vultur gryphus), Cattle egret (Bubulcus ibis), Saddle-billed stork (Ephippiorhynchus senegalensis), White stork (Ciconia ciconia), Great cormorant (Phalacrocorax carbo)
Sphenisciformes
(2 species)
Little [Fairy] penguin (Eudyptula minor), Humboldt penguin (Spheniscus humboldti)
Eurypygiformes
(1 species)
Sunbittern (Eurypyga helias)
Charadriiformes
(10 species)
Banded lapwing (Vanellus tricolor), Spur-winged Lapwing (Vanellus spinosus), Beach stone-curlew (Esacus magnirostris), Bush stone-curlew (Burhinus grallarius), Lesser black-backed gull (Larus fuscus), Black-headed gull (Chroicocephalus ridibundus), European herring gull (Larus argentatus), Kelp [Dominican gull] (Larus dominicanus), Silver gull (Chroicocephalus novaehollandiae), Greater crested tern (Thalasseus bergii), Painted button-quail (Turnix varius)
Gruiformes
(7 species)
Brolga (Grus rubicunda), Grey crowned crane (Balearica regulorum), Dusky moorhen (Gallinula tenebrosa), Red-crowned crane [Japanese crane] (Grus japonensis), Eurasian coot (Fulica atra), Purple swamphen (Porphyrio porphyrio)
Caprimulgiformes
(1 species)
Tawny frogmouth (Podargus strigoides)
Otidiformes
Australian bustard (Ardeotis australis)
Musophagiformes
(5 species)
Guinea [Green, Buffon’s] turaco (Tauraco persa), Ross's [Lady Ross's] Turaco (Musophaga rossae), White-cheeked turaco (Tauraco leucotis), Red-crested turaco (Tauraco erythrolophus), Western plantain-eater (Crinifer piscator)
Cuculiformes
(2 species)
Channel-billed cuckoo (Scythrops novaehollandiae), Pacific [Eastern] koel (Eudynamys orientalis),
Columbiformes
(18 species)
Mindanao Bleeding-heart [Bartlett's Bleeding Heart Dove] (Gallicolumba crinigera), Crested pigeon (Ocyphaps lophotes), Diamond dove (Geopelia cuneata), Common emerald dove (Chalcophaps indica), Pied imperial pigeon (Ducula bicolor), Nicobar pigeon (Caloenas nicobarica), Rock pigeon (Columba livia), Rose-crowned fruit-dove (Ptilinopus regina), Superb fruit dove [Purple-crowned fruit dove] (Ptilinopus superbus), Victoria crowned pigeon (Goura victoria), White-headed pigeon (Columba leucomela), Spotted Dove (Spilopelia chinensis), White-breasted Ground Dove [White-bibbed Ground Dove, Purple Ground Dove] (Gallicolumba jobiensis), Wonga pigeon (Leucosarcia melanoleuca), Wood pigeon (Columba palumbus), Spinifex pigeon (Geophaps plumifera), Zebra dove [Barred Ground Dove] (Geopelia striata), Speckled pigeon [African rock pigeon] (Columba guinea)
Phoenicopteriformes
(2 species)
American flamingo (Phoenicopterus ruber), Greater flamingo (Phoenicopterus roseus)
Podicipediformes
(1 species)
Great crested grebe (Podiceps cristatus)
Neognathae, Galloanseres
Galliformes
(17 species)
Golden pheasant [Chinese pheasant] (Chrysolophus pictus), Brown quail [swamp quail] (Coturnix ypsilophora), Chestnut-backed buttonquail (Turnix castanotus), King quail (Coturnix chinensis or Excalfactoria chinensis), Stubble quail (Coturnix pectoralis), Indian peafowl [blue peafowl] (Pavo cristatus), Brush turkey (Alectura lathami), Domestic fowl (Gallus gallus domesticus), Red junglefowl (Gallus gallus), Great Argus pheasant (Argusianus argus), Helmeted guineafowl (Numida meleagris), Green peafowl [Java peafowl] (Pavo muticus), Vulturine guineafowl (Acryllium vulturinum), Lady Amherst's pheasant (Chrysolophus amherstiae), Nocturnal curassow (Nothocrax urumutum), Siamese fireback [Diard's fireback] (Lophura diardi), Temminck's tragopan (Tragopan temminckii)
Anseriformes
(20 species)
African Pygmy Goose (Nettapus auritus), Blue-billed duck (Oxyura australis), Ringed teal (Callonetta leucophrys), Chestnut teal (Anas castanea), Knob-billed [comb] duck (Sarkidiornis melanotos), Mallard female (Anas platyrhynchos), Wandering whistling duck (Dendrocygna arcuata), Black swan (Cygnus atratus), Egyptian goose (Alopochen aegyptiaca), Fulvous whistling duck (Dendrocygna bicolor), Greylag goose (Anser anser), Magpie goose (Anseranas semipalmata), Muscovy duck (Cairina moschata), Hawaiian goose [Nene] (Branta sandvicensis), Red crested pochard (Netta rufina), Raja Shelduck [Burdekin duck] (Tadorna radjah), Tufted duck (Aythya fuligula), Canada goose (Branta canadensis), Cape Barren goose (Cereopsis novaehollandiae), Mandarin duck (Aix galericulata)
Palaeognathae
Struthioniformes
(1 species)
Common ostrich (Struthio camelus)
Casuariformes
(2 species)
Southern Cassowary (Casuarius casuarius), Emu (Dromaius novaehollandiae)